COMBUSTION
for cleaner fuels and better catalysts
To formulate the fuel of the future, IRP3 looks at new molecules that can be produced within the techno-economic constraints of a refinery and that have the potential to reduce pollutant emissions when added to fossil-derived fuels.
This research will help to identify the best fuels (or fuel mixtures) for low-emission energy conversion, and to design and manufacture optimised, cost-effective nanostructured materials for catalysis.
FEATURED RESEARCH
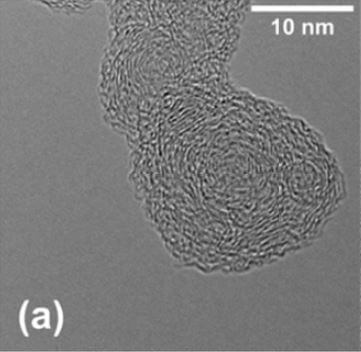
Studying soot nanostructure using HRTEM
The nanostructure of soot was studied using high-resolution transmission electron microscopy (HRTEM). The samples were collected in an n-heptane laminar co-flow diffusion flame doped with cyclohexene (CHE) and cyclopentene (CPE). HRTEM images show an amorphous core and a shell composed by graphitic-like domains are also present – the graphitic-like domains are formed by the graphitisation or annealing of the particles at high temperature. The image analysis results allow calculation of parameters such as fringe length, fringe
tortuosity and inter-fringe spacing. It was observed that most of the fringes present a low degree of curvature. Nevertheless, the addition of
cyclopentene to n-heptane flames increases the amount of highly curved fringes. This has applications for understanding the soot formation process and the effect of biofuels on reducing pollutant formation.
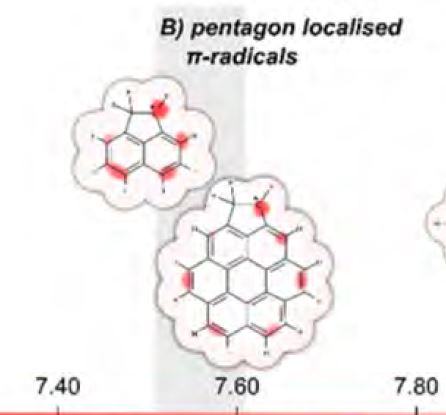
Modelling chemical mechanisms
CARES researchers are continuing to explore the formation of soot in flames as well as the nanostructure of carbon materials. Experiments have now demonstrated that curvature can be integrated into soot precursor molecules and this increases the rate of nucleation in diffusion flames. They are now developing the ability to increase the ionic concentration in flames using a plasma injector and are planning experiments that will be able to determine the influence of ionic species on nucleation.
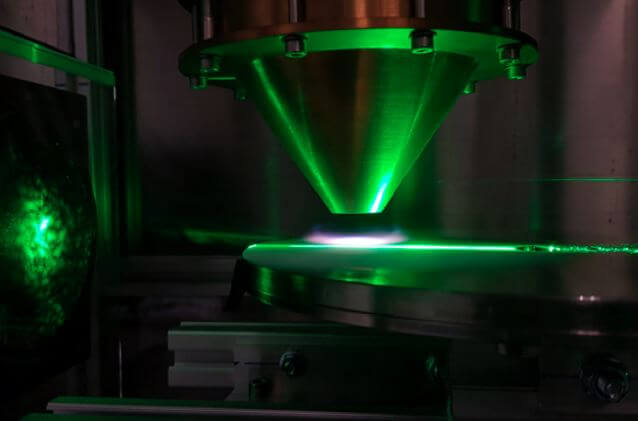
Modelling crystal nanostructures
Researchers have looked at how various crystal structures of TiO2 nanoparticles are formed in flame synthesis. The study was based on new experimental measurements of the crystal phase composition of TiO2 prepared in premixed stagnation flames with varying flame dilution ratio. The particle formation was modelled with a detailed particle model to test different hypotheses of crystal phase formation. It was found that the conventional hypothesis of crystal phase thermodynamic stability based on surface energies alone does not sufficiently
explain the experimental observations. It was proposed that the incipient particle composition is the key factor controlling the crystal phase
formation in a flame synthesis condition.